Veröffentlicht: 10.03.2025. Rubrik: Fantastisches
Terraforming Deserta Umbrae (blueprint Mars)
‘Deserta Umbrae’ is a desert planet with a thin CO₂ atmosphere, high UV radiation, minimal water and nitrogen-poor soil. Deserta Umbrae has a thin atmosphere (about 0.6% of Earth's pressure, about 6 hPa), little to no magnetic field (radiation protection is absent), cold temperatures (-80 °C on average), and CO₂-rich soils with ice deposits (regolith, polar ice caps). Our tools: a compact probe with master AI, mini AI beetles (self-learning, ant level) and chemo-synthetic microorganisms. Alternatively, we use digital nanorods in symbiosis with microorganisms.
Our probes land in regions with resources (e.g. ice, metal oxides in the regolith). The mini-bugs (size: mm range, with drill/sensors) start mining iron, silicon and other metals. They adapt to the conditions (e.g. dust storms) and optimise their efficiency through self-learning.
The master AI prioritises the construction of larger machines (e.g. 3D printers that produce nanorods or small reactors). Our little KI-Ant-Beetles could assemble micro-factories that produce materials for atmosphere modification (e.g. greenhouse gases).
Without a magnetic field, solar energy is limited by radiation and dust storms. The AI could build mini-reactors (e.g. radioisotope generators) that run on local uranium/thorium or synthesise methane from CO₂ and ice (Sabatier reaction: CO₂ + 4H₂ → CH₄ + 2H₂O).
The machines process ice and regolith to release CO₂ (abundant in Martian -sorry, uh Deserta Umbrae's- soils) and water vapour. AI beetles could produce metal nanorods (e.g. 9 µm long, made of iron) that are sprayed into the atmosphere to enhance the greenhouse effect.
In parallel, we use microorganisms that convert CO₂ and H₂ (from ice) into CH₄ (methane) – a potent greenhouse gas. Chemo-synthesis (e.g. methanogenesis: CO₂ + 4H₂ → CH₄ + 2H₂O) does not require light, which makes it ideal for the low-light conditions on Deserta Umbrae.
The magnetic field is, of course, a problem because the atmosphere is constantly escaping. Until a final technical solution emerges, the only option is to continuously refill the atmosphere using microbes/automata.
Our microbes (e.g. extremophile archaea) use local resources (CO₂, H₂, sulfates) and develop in an environmentally specific way (genetically adaptable via synthetic biology). They form biofilms under the surface (radiation protection) and release O₂.
The master AI monitors the evolution, selects successful strains and promotes hybrids that fix N₂ (necessary for plant growth). Our goal here is a primitive flora (e.g. algae-like) that makes the soil fertile. The robots melt ice (via methane release) and create small water cycles. Microbes could break down salts (perchlorates) to make water usable. The master AI monitors the evolution, selects successful strains and promotes hybrids that fix N₂ (necessary for plant growth).
The effect of UV radiation on microorganisms is another problem, but microbes could form silicate or metal-based shields (e.g. SiO₂ layers) as a by-product of their chemo-synthesis, by absorbing silicon from the regolith and secreting it as a protective layer.
So: Genetically modified metabolic pathways – e.g. SiO₂ precipitation from dissolved silicate (Si(OH)₄ → SiO₂ + 2H₂O) as a waste product. Energy from chemo-synthesis (CO₂ + H₂ → CH₄); biofilms with SiO₂ layers provide sub-surface protection, reflect UV radiation and stabilise soils. Likewise, our microbes could release N₂ to build up the atmosphere. Mars regolith contains nitrogen compounds (e.g. nitrates, NH₄⁺), which they break down; denitrification (NO₃⁻ → N₂ + O₂) or ammonia oxidation (NH₃ → N₂ + H₂O). Energy from chemo-synthesis (e.g. sulfate reduction). N₂ serves as a buffer gas and is less volatile than CO₂. On top of that, O₂ is a bonus for the later flora.
Let us now turn to our ant-bug design and its energy supply. The machines are powered by mini-reactors (e.g. with U-235 or Th-232 from regolith). The size of our beetles is in the mm range (e.g. 5 mm), scalable to cm for larger machines. Our machines are equipped with diamond drills, sensors (UV, metal detection), microprocessors (self-learning, beetle level), energy (mini-fission via U-235 pellets).
Let us now turn to the nanorods: these are helical nanotubes (e.g. made of gold, silicon or carbon) with bioactive coatings (peptides) to attract microbes. They are designed as machines with Turing-like computability that can solve any task. They should be 10-100 nanometres in size, i.e. small enough for microbial interaction (e.g. chemo-synthesis in ‘Deserta’), but large enough for internal logic. We use photovoltaics and/or chemical energy (e.g. oxidation of microbial waste) as energy sources. Individual nanorods are therefore, so to speak, ‘cells’ with basic computing power (e.g. state transitions like finite state machines), which combine to form ‘organs’ (structures).
Hardware considerations:
Core: quantum dot (qubit-like) for simple calculations – e.g. states ‘0’, ‘1’, ‘superposition’ (inspired by quantum computing).
Shell: DNA origami structure (see DNA nanotech research), flexible for self-assembly.
Sensors: infrared/ultrasound for environmental perception (e.g. other nanorods, microbes).
Actuators: magnetic tips or chemical ‘glue’ (e.g. van der Waals forces) for docking.
With regard to the software, we use a kind of ant algorithm to generate an exponentially increasing swarm intelligence without central control (local rules). We use a simple neural network consisting of three layers (input, hidden, output) that is trained by feedback from the environment. The aim is self-organisation into larger structures. The following mechanisms could be used:
Rule 1: ‘Search for neighbours’ – nanorods dock when they receive similar signals (e.g. UV frequencies).
Rule 2: ‘Form chains’ – linear nanorod compounds (e.g. like termite mounds) for simple tasks (e.g. microbial transport).
Rule 3: ‘Form networks’ – chains combine to form 2D/3D lattices (e.g. helix or cube) when the task becomes more complex (e.g. O₂ release).
Individual nanorods are ‘dumb’ (e.g. they only react to light gradients), but in the swarm ‘intelligence’ arises through feedback. A rather utopian goal here is a self-optimising ‘superorganism’ that, for example, terraforms Deserta Umbrae.
Another possibility would be a symbiotic system with microorganisms in which various nanorod structures serve as a ‘mycelium’ scaffold for microbes. The structures ‘feed’, protect and stimulate integrated microbes.
A rough analysis of the terraforming process could be as follows:
Step 1: Sowing: Nanorods and microbes distributed by spaceship (e.g. dust clouds over craters).
Step 2: Network formation: Helical chains → sponges protect microbes, start O₂ production.
Step 3: Soil formation: nets ‘weave’ microbes into sand, humus is created, N₂ is released.
Step 4: Scaling: billions of nanorods form a planet-wide ‘mycelium’ and/or ‘superorganism’.
A fleet of 5 ships (3 as a core fleet, 2 redundant) should be used. Specialisation would be desirable, e.g.:
Ship 1: Nanorod storage – 10 m long, 5 m wide, 500 kg Nanorods (10¹⁵ units).
Ship 2: Microbe tanks – 15 m long, 8 m wide, 1000 L microbe cultures (e.g. cyanobacteria).
Ship 3: Control drone – 5 m long, 3 m wide, AI for coordination (seeding pattern).
If available, ion thrusters should be used or whatever. Now Aldhar Ibn Beju has had enough and lets you think for a while.
© 2025 Q.A.Juyub alias Aldhar Ibn Beju

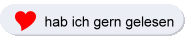